What is your brain doing when you rest?
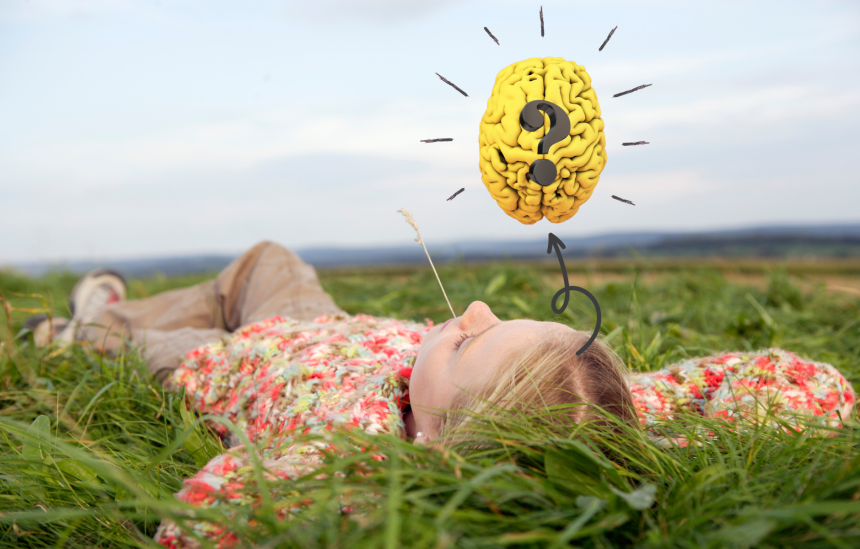
When you are doing nothing, thinking of nothing, and letting your brain have a rest, does your brain really “turn off”?
In 1995, biomedical engineer Biswal published a paper addressing this question. He was analyzing functional magnetic resonance imaging (fMRI) [1, 2]. He was trying to remove the noise caused by the movements associated with respiration and heartbeat from the signal representing actual brain activity. He analyzed the correlation between the fMRI signal in the motor cortex and other brain regions. To his surprise, he found that the motor cortex was functionally connected to other motor-related brain regions, even when a person was at rest [2]. Functional connection means that when one brain region is activated or engaged, the other region shows a similar pattern of activation or engagement. The regions can be thought of as “coupled”. This connectivity (or coupling) is known as resting-state functional connectivity (RSFC). This finding challenged the prevailing notion that brain activity was primarily driven by external tasks or stimuli. The discovery of RSFC opened up new possibilities for studying the brain’s intrinsic functional architecture.
In 2001, Raichle and colleagues at Washington University School of Medicine described the activity of brain regions that were more active at rest as a baseline state, or a “default mode” of the brain [3]. This was based on the repeated observation that regions forming this network exhibited reduced metabolic activity when people are engaged in cognitive tasks—these observations were made using a technique called Positron Emission Tomography (PET), which can measure metabolic activity using radioactive substance. In 2003, Greicius and colleagues identified brain regions with greater activity at rest and named them the default mode network (DMN). The results were consistent with the work of Raichle and colleagues referred to as the “default mode” baseline [4].
The DMN exhibits higher activity when the brain is at rest, rather than engaged in specific cognitive, motor or language tasks, such as auditory discrimination and speech processing. As shown in figure 1, this network includes the following brain areas: the orbital frontal cortex, the medial prefrontal cortex (MPFC), the anterior cingulate cortex (ACC), the lateral temporal cortex, the inferior parietal lobe, the posterior cingulate cortex (PCC), the retrosplenial cortex, and structures such as the hippocampus and parahippocampal gyrus. The robustness of the DMN across studies and the ease with which it is observed have contributed to spurring the acceptance and utilization of resting-state fMRI as a rigorous neuroscience tool. The number of publications about RSFC took off in the mid-2000s and now thousands of papers are being published each year using this technique. Resting-state fMRI has become a widely used tool in neuroscience research.
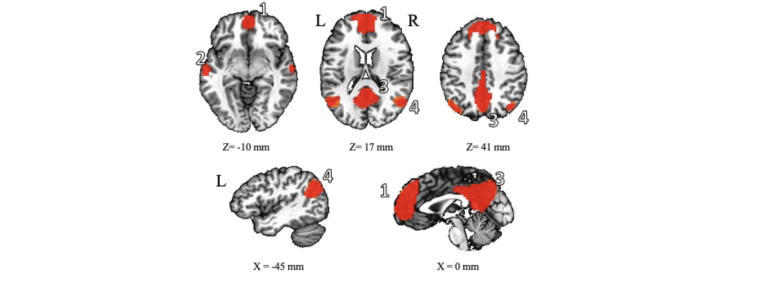
Figure 1. The default mode network (DMN) in humans, shown here in axial (top row) and sagittal (bottom row) views: significant clusters include 1, medial prefrontal cortex (MPFC)/anterior cingulate cortex (ACC); 2, lateral temporal cortex; 3, posterior cingulate cortex (PCC)/retrosplenial cortex; 4, inferior parietal lobe; 5, hippocampus/parahippocampal cortex. [5, 6].
While the precise functions of the DMN are not yet fully understood, research suggests that it may be supporting various self-referential cognitive processes, such as self-reflection, introspection, mental time travel (recollecting the past and envisioning the future), and daydreaming. Moreover, studies have found that DMN exhibit abnormalities in many neuropsychiatric and neurological disorders, such as depression, anxiety, autism, and Alzheimer’s disease, suggesting that functional connectivity may be an index of brain health. As such, research on DMN holds significant potential for understanding and treating these conditions.
References:
- Biswal, B., et al., Functional connectivity in the motor cortex of resting human brain using echo-planar mri. Magnetic Resonance in Medicine, 1995. 34(4): p. 537-541.
- Biswal, B.B., Resting state fMRI: a personal history. Neuroimage, 2012. 62(2): p. 938-44.
- Raichle, M.E., et al., A default mode of brain function. Proceedings of the National Academy of Sciences, 2001. 98(2): p. 676-682.
- Greicius, M.D., et al., Functional connectivity in the resting brain: A network analysis of the default mode hypothesis. Proceedings of the National Academy of Sciences, 2003. 100(1): p. 253-258.
- Raichle, M.E., The Brain’s Default Mode Network. Annual Review of Neuroscience, 2015. 38(1): p. 433-447.
- Lu, H., et al., Rat brains also have a default mode network. Proceedings of the National Academy of Sciences, 2012. 109(10): p. 3979-3984.
- Farras-Permanyer, L., et al., Age-related changes in resting-state functional connectivity in older adults. Neural Regeneration Research, 2019. 14(9): p. 1544-1555.
- Ferreira, L.K. and G.F. Busatto, Resting-state functional connectivity in normal brain aging. Neuroscience & Biobehavioral Reviews, 2013. 37(3): p. 384-400.
- Grady, C.L., O. Grigg, and C. Ng, Age differences in default and reward networks during processing of personally relevant information. Neuropsychologia, 2012. 50(7): p. 1682-1697.
- Damoiseaux, J.S., et al., Reduced resting-state brain activity in the “default network” in normal aging. Cerebral Cortex, 2008. 18(8): p. 1856-1864.
- Maguire, E.A., et al., Navigation-related structural change in the hippocampi of taxi drivers. Proceedings of the National Academy of Sciences, 2000. 97(8): p. 4398-4403.