History of Transcranial Magnetic Stimulation
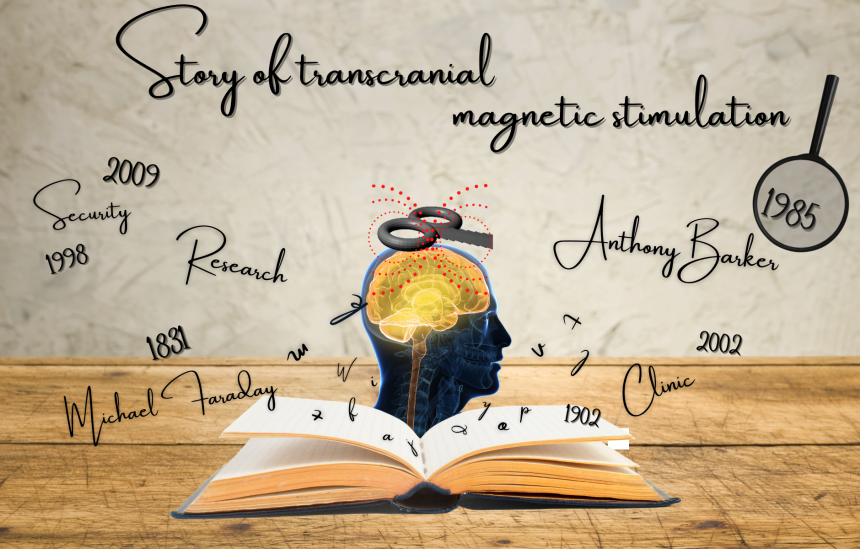
When was the first use of transcranial magnetic stimulation (TMS)?
The principle of electromagnetic induction underlying the operation of TMS was discovered in 1831 by the English physicist Michael Faraday (Faraday, 1839). This principle states that a rapidly changing magnetic field produces an electric current in a nearby conductive element (Irving and Chang, 2015). In the case of TMS, a magnetic field is generated by a current flowing through a plastic coil positioned on the scalp (see figure 1). The magnetic field in turn induces a secondary current in a small area of the brain located under the coil, thus acting on the activity of neurons in brain regions located under the coil (for more information on how TMS works, we invite you to read our blog post “TMS: for a better understanding”).
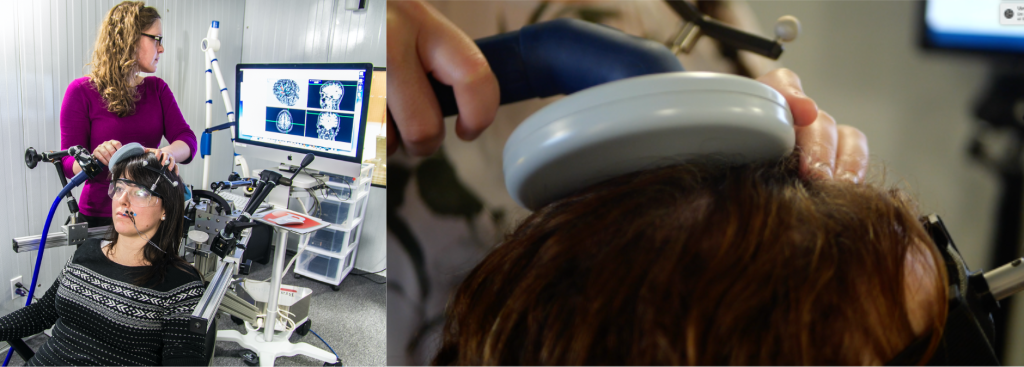
Figure 1. Illustration of a TMS session, in which the coil can be seen positioned on the participant’s head.
In 1896, Jacques-Arsène d’Arsonval, a French physicist, was perhaps the first to document a technique akin to modern TMS (Georges et al., 2007). Arsonval observed that when individuals positioned their heads on a coil emitting a magnetic field, they reported phosphenes (i.e., the sensation of seeing a light or perceiving spots in the visual field), a phenomenon today associated with TMS-induced activity in the retina or visual cortex, such as when stimulation is applied to the visual cortex, i.e., the part of the brain that receives visual information (Webster and Ro, 2017). Arsonval also induced vertigo and syncope with his method (Geddes, 1999).
Following the work of Arsonval, in 1902 in Austria, psychiatrists Adrian Pollacsek and Berthold Beer reported using an electromagnetic coil, positioned above the head to treat depression and neuroses. This experiment would be one of the first attempts to use a technique similar to TMS for clinical purposes (Georges et al., 2007).
A few decades later, in 1959, Alexander Kolin and his colleagues, working in the department of biophysics at the University of California in the United States, were the first to trigger a muscle contraction in the sciatic nerve of a frog following magnetic stimulation of the sciatic nerve (Kolin et al., 1959). This experiment was clear evidence that a magnetic field could induce enough current to stimulate a motor nerve.
However, it was not until 1985 that the first modern TMS device was used in clinics and research. This device was developed by researcher and medical physicist Anthony Barker and his team at the University of Sheffield in England. It was this team that first documented hand movements induced by TMS and the electrical activity associated with it (Barker et al., 1985). The team received an innovation award from the Institute of Electrical Engineers, in 1987, for the development of the TMS (Walsh and Pascual-Leone, 2003).
The first investigations using TMS were carried out on people with multiple sclerosis or motor neuron diseases and aimed to measure the speed of propagation of nerve impulses between the motor cortex and the muscles, for diagnostic purposes (Horvath et al., 2010). The first TMS devices had some limitations. The charging time was long, and the device tended to overheat when the magnetic pulses were close in time (Noohi and Amirsalari, 2016). With the growing enthusiasm for TMS, several private companies have now developed and marketed more efficient TMS devices, which can be used with more rapid protocols including repetitive TMS protocols (a type of TMS where pulses or bursts of pulses are applied at intervals of a few seconds or milliseconds; Walsh and Pascual-Leone, 2003; Noohi and Amirsalari, 2016). Coils of different shapes have also been developed (see figure 2), making it possible to vary the spatial resolution of the TMS (Georges et al., 2007).
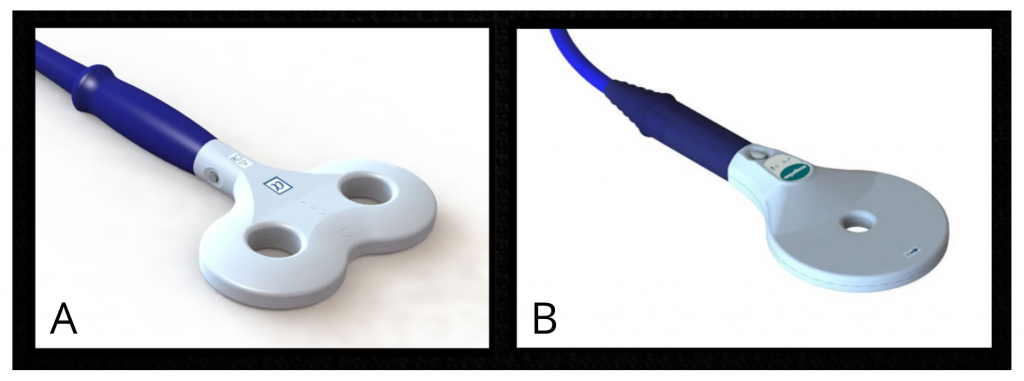
Figure 2. Examples of two types of coils. A) Eight-shaped coil. B) Circular coil. Source: Magstim (2021).
In 1991, one of the first scientific papers reporting the use of repetitive TMS was published by Alvaro Pascual-Leone, now a professor of neurology at Harvard Medical School, and his colleagues. In this study, the research team observed that the application of repetitive TMS to regions of the left hemisphere of the brain could interrupt speech production, a phenomenon sometimes referred to as a “speech arrest” (Horvath et al., 2010). A few years later, in 1995, Kolbinger and his colleagues, affiliated with universities in Germany and Austria, were among the firsts to publish the results of a proof-of-concept study (i.e., a study aimed at showing the feasibility of an innovation) in which repetitive TMS was used in people with major depression refractory to pharmacological treatments. A reduction in symptoms was observed following the TMS treatment (Kolbinger et al., 1995).
In the late 1990s, research teams combined TMS with neuroimaging techniques such as magnetic resonance imaging (MRI) and positron emission tomography (Horvath et al., 2010). These studies showed that TMS affected brain activity directly underneath the coil, but also in nearby regions, though with a reduced magnitude (e.g., Paus et al., 1997).
From the beginning of the use of TMS, several ethical considerations have been raised. These considerations included the safety of TMS devices, as well as potential long-term side effects (Horvath et al., 2010). These considerations led to the publication of the first safety guidelines for the safe usage of TMS in 1998 by Wassermann (Horvath et al., 2010). Since that time, other international safety guidelines have been published and then revised to minimize the risks associated with TMS (Rossi et al., 2009 and 2020). These guidelines are rigorously followed by the members of our laboratory, which are also trained by Dr. Pascale Tremblay on the principles and safety of TMS.
Over the years, TMS has proven to be an important research tool in the field of neuroscience and psychiatry, to study the functioning of the brain and spinal cord in healthy people or people with neurological or psychiatric disorders. It is a tool that we use in our laboratory to understand how the brain allows us to perceive and produce speech. On the clinical side, repetitive TMS was approved in 2002 by Health Canada. It was also approved by the US Food and Drug Administration (FDA) for the treatment of drug-resistant depression in 2008, migraines in 2013, and obsessive-compulsive disorder in 2018 (Horvath et al., 2011; Stultz et al., 2020). TMS is used as a therapeutic tool in the clinic, since it can induce medium—or long-term changes in the excitability and connectivity of neural networks (Ziemann, 2017), a phenomenon called “plasticity induced by experience.”
Finally, research is still ongoing to better understand how TMS affect brain activity, its mechanism of action. One approach is to TMS with neuroimaging techniques such as electroencephalography, or mathematical modelling techniques, making it possible to visualize the distribution of the electric field following stimulation. Hence, the history of the TMS is still being written!
Further readings:
References:
Barker AT, Jalinous, R., & Freeston, IL (1985). Non-invasive magnetic stimulation of human motor cortex. Lancet, 1 (8437):1106-7.
Faraday F. (1839). Experimental research in electricity. Volume 1. Taylor and Francis, London.
Geddes, LA (1999). Retrospectroscope . d’Arsonval, physician and inventor. IEEE Engineering in Medicine and Biology, 18 (4), 118-122.
George, MS, Bohning , DE, Loberbaum , JP, Nahas , Z., Anderson, B., Borckardt , JJ, … Belmaker, RH (2007). Overview of transcranial magnetic stimulation. In MS George, & RH Belmaker (Eds.), Transcranial magnetic stimulation in clinical psychiatry. Washington, DC: American Psychiatric Publishing, Inc.
Horvath, JC, Perez, JM, Forrow, L., Fregni, F., & Pascual-Leone, A. (2011). Transcranial magnetic stimulation: A historical evaluation and future prognosis of therapeutically relevant ethical concerns. Journal of Medical Ethics: Journal of the Institute of Medical Ethics, 37 (3), 137–143.
Irving, MR, & Chang, AD (2015). Introduction to brain stimulation. In Irving, MR (Ed.), Brain stimulation: Methodologies and Interventions. Hoboken, NJ: John Wiley & Sons, Inc.
Kolbinger , HM, Höflich , G., Hufnagel, A., Müller, H.-J., & Kasper, S. (1995). Transcranial magnetic stimulation (TMS) in the treatment of major depression: A pilot study. Human Psychopharmacology: Clinical and Experimental, 10 (4), 305–310.
Kolin, A., Brill, NQ, & Broberg, PJ (1956). Stimulation of irritable tissues by means of an alternating magnetic field. Proceedings of the Society for Experimental Biology and Medicine, 102 :251–253.
Paus, T., Jech, R., Thompson, CJ, Comeau, R., Peters, T., & Evans, AC (1997). Transcranial magnetic stimulation during positron emission tomography: A new method for studying connectivity of the human cerebral cortex. The Journal of Neuroscience, 17 (9), 3178–3184.
Rossi, S., Antal, A., Bestmann, S., Bikson, M., Brewer, C., Brockmöller, J. … Hallet, M. (2020). Safety and recommendations for TMS use in healthy subjects and patient populations, with updates on training, ethical and regulatory issues: Expert guidelines. Clinical Neurophysiology, 132 (1):269-306.
Rossi, S., Hallett, M., Rossini, PM, Pascual-Leone, A., & The Safety of TMS Consensus Group. (2009). Safety, ethical considerations, and application guidelines for the use of transcranial magnetic stimulation in clinical practice and research. Clinical Neurophysiology, 120 (12), 2008–2039.
Stultz, DJ, Osburn, S., Burns, S., Pawlowska-Wajswol, S., & Walton, R. (2020). Transcranial magnetic stimulation (TMS) safety with respect to seizures: A literature review. Neuropsychiatric Disease and Treatment, 16 :2989-3000.
Walsh, V., & Pascual-Leone, A. (2003). Transcranial Magnetic Stimulation: A Neurochronometrics of Mind. MIT press, Cambridge, MA, 297 p.
Webster, K., & Ro, T. (2017). Retinal and visual cortex distance from transcranial magnetic stimulation of the vertex affects phosphene perception. Experimental Brain Research, 235 (9), 2857–2866.
Ziemann, U. (2017). Thirty years of transcranial magnetic stimulation: where do we stand? Experimental Brain Research, 235 (4), 973-984.