Synaptic transmission
How are nerve impulses transmitted from one neuron to another? What are the mechanisms allowing the transmission of signals at the level of the synapse?
In a previous blog post, we told you about the anatomy and functioning of neurons. We mentioned that synapse is a contact area between neurons and that, in most cases, there is no direct contact between neurons. This is the case for chemical synapses (see figure 1)—the majority in the nervous system—where information is transmitted from one neuron to another by means of chemical molecules called neurotransmitters. The transmission of neurotransmitters between neurons involves a series of steps which we provide an overview of in this post.
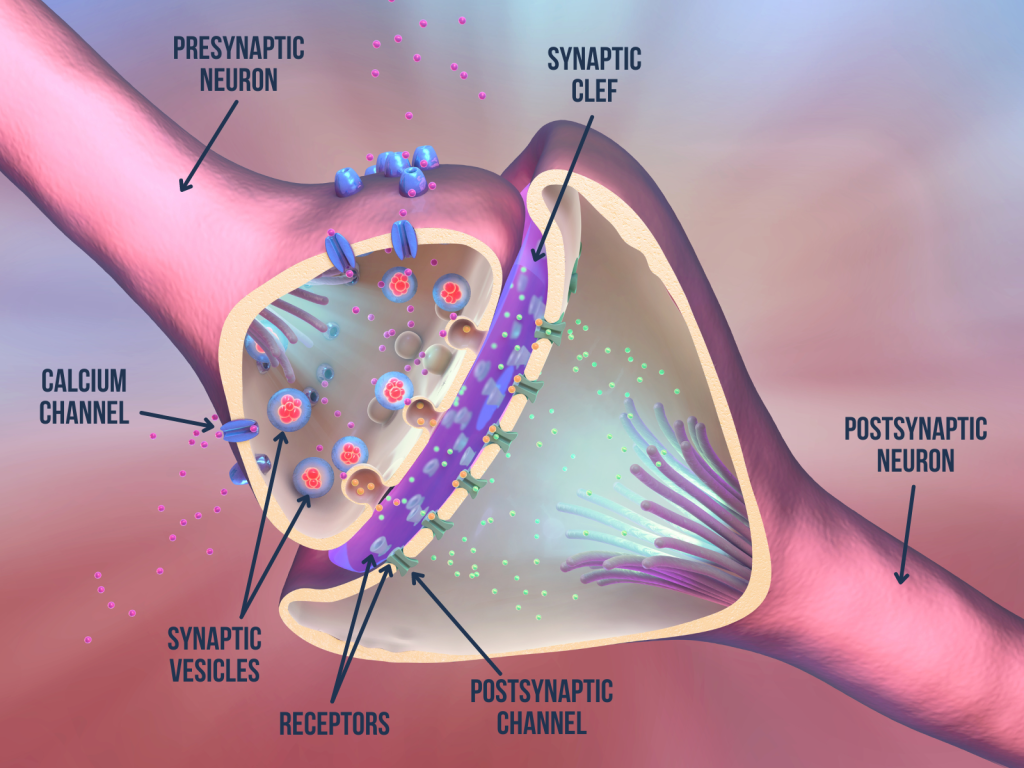
Figure 1. Illustration of a chemical synapse. Adapted from 3d Illustration of the scientific function of a synapse or neuronal connection with a nerve cell by man_at_mouse on Canva.
Stages of signal transmission at chemical synapses (Purves et al., 2015):
- Arrival of an Electrical Signal
The triggering event is the arrival of an electrical signal (called an action potential) in the terminal buttons of the presynaptic neuron, i.e., the neuron that transmits the message.
2. Entry of Calcium (Ca2+) in the Terminal Buttons
This electrical signal causes the opening of voltage-sensitive calcium channels located in the membrane of the terminal buttons (see figure 1). Their opening allows the rapid entry of Ca2+ (illustrated by the pink balls in the figure) into the terminal buttons of the presynaptic neuron.
3. Fusion of Synaptic Vesicles and Release of Neurotransmitters
Synaptic vesicles (akin to small bags) containing the neurotransmitters are synthesized by the neuron then stored in the terminal buttons. When the concentration of Ca2+ increases in the terminal buttons, a phenomenon of exocytosis occurs, i.e., the synaptic vesicles fuse with the membrane of the presynaptic neuron. This fusion results in the release of neurotransmitters into the synaptic cleft (see Figure 1, where neurotransmitters are shown in red/orange).
4. Binding of Neurotransmitters to Receptors and Opening of Postsynaptic Channels
Neurotransmitters diffuse into the synaptic cleft and bind to receptors in the membrane of the post-synaptic neuron (the neuron that receives information). The binding of neurotransmitters causes the opening or closing of channels located in the membrane of the post-synaptic neuron, depending on the type of neurotransmitter. Indeed, there is a great diversity of neurotransmitters (there are hundreds of them!). Some are excitatory and some are inhibitory (i.e., some neurotransmitters can promote propagation of nerve impulses, while others inhibit propagation). Dopamine, serotonin, norepinephrine, glutamate, GABA, acetylcholine and endocannabinoids are some examples of neurotransmitters. Neurotransmitters have specialties. For example, acetylcholine is the main neurotransmitter used in the motor system (which specializes in movement control) and muscle fibres. In short, the opening or closing of the post-synaptic channels caused by the binding of neurotransmitters modifies the capacity of the ions (shown in green in figure 1) to cross the membrane of the post-synaptic neuron. The conductance of the post-synaptic neuron is then modified, which increases or reduces its probability of emitting an electrical signal and in turn transmitting the nerve impulse to other neurons.
5. Elimination or Degradation of Neurotransmitters and Recycling of Vesicles
The residual neurotransmitters are either destroyed or recaptured by the presynaptic neuron or by glial cells. After the neurotransmitters are released into the synaptic cleft, the synaptic vesicles that contained them are recycled for reuse.
It is important to mention that the same neuron can release different types of neurotransmitters. Moreover, since a single neuron can receive information from thousands of other neurons via its synapses, it is the sum of the conductance changes in the post-synaptic neuron that will determine whether or not this neuron will emit an electrical signal. This phenomenon is called the “summation of postsynaptic potentials” (Purves et al., 2015).
In the nervous system, in addition to chemical synapses, there are also electrical synapses. These are abundant in the developing nervous system, but are less common than chemical synapses in the adult nervous system. Electrical synapses are found in several regions of the central nervous system, including the retina, olfactory bulbs, hypothalamus, cerebellum, and brainstem (Meriney & Fanselow, 2019; Purves et al., 2015). Electrical synapses allow electrical signals to pass directly from one neuron to another, through gap junctions, which are specialized channels allowing direct contact between neurons (as opposed to chemical synapses, for which there is no direct contact between neurons). Another distinction between chemical and electrical synapses concerns the direction of information flow. In chemical synapses, the transmission of information is unidirectional: the information circulates from the presynaptic neuron to the post-synaptic neuron. In contrast, in electrical synapses, the exchange of information can be bidirectional. Electrical synapses have the advantage of allowing groups of neurons to have synchronized electrical activity, essential for rhythmic functions such as breathing, which is regulated by the brainstem (Purves et al., 2015).
In our laboratory, we use a brain stimulation technique that temporarily modifies the transmission of information between neurons, namely transcranial magnetic stimulation (TMS). How TMS can alter synaptic transmission and brain function will be the subject of another post! Forthcoming!
For more detailed information on synaptic transmission and neurotransmitters, we encourage you to consult the following book:
Purves, D., Augustine, GJ, Fitzpatrick, D., Hall, WC, LaMantia, AS, & White, LE (Eds). (2015). Neurosciences (5th ed.). Sinauer Associates.
Further readings:
References:
Meriney, SD, & Fanselow, EE (2019). Electrical Synapses. In Meriney, SD, and Fanselow, EE (eds.), Synaptic transmission (1st ed., p. 65-91). Academic Press.
Purves, D., Augustine, GJ, Fitzpatrick, D., Hall, WC, LaMantia, AS, & White, LE (2015). Neuroscience (5th ed.). Sinauer Associates.